This Fox Chase professor participates in the Undergraduate Summer Research Fellowship.
Learn more about Research Volunteering.
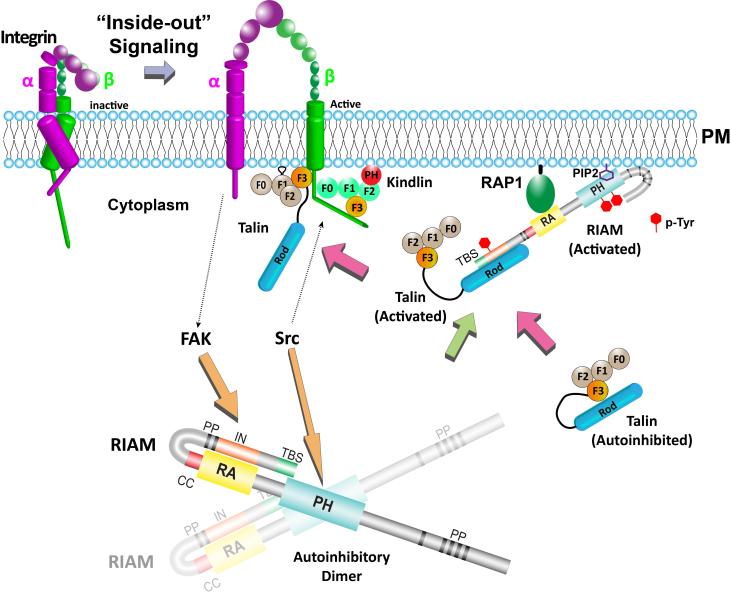
This Fox Chase professor participates in the Undergraduate Summer Research Fellowship.
Learn more about Research Volunteering.
Professor
A postdoctoral position is available immediately at Fox Chase Cancer Center (Philadelphia, PA) for a highly-motivated individual in the field of structural biology and protein biochemistry.
Our lab aims to understand the molecular mechanisms underlying the signaling events mediated by integrins, small GTPases, as well as kinases, which have strong implications in cancer progression, metastasis and other human diseases, and to translate this knowledge into inhibitor/drug discovery. For this, we employ a combination of techniques including protein crystallography, electron microscopy, binding kinetics analysis, functional assays in mammalian cells, and genetic analysis. Our study will facilitate efforts to discover novel targets for therapeutic intervention of immune system disorders, tumor metastasis, and many other related diseases.
The candidate is expected to have strong experience in X-ray crystallography, protein biochemistry, and molecular biology. Prior experiences in mammalian cell culture, flow cytometry, drug screen, and cancer biology, are desired but not required. Fox Chase Cancer Center is a long-standing NCI-designated Comprehensive Cancer Center with multidisciplinary research interests from basic biological sciences to drug development and clinical studies. The institute is located in a quiet, safe, friendly neighborhood at the outskirts of the city. It is one of the top-ranked work places for visiting scholars and postdoctoral researchers. We also offer an outstanding research environment and strong support for the career development for our scientists.
Please send a cover letter describing previous research experience and future plans, a current CV, a copy of graduate transcript, and a list of three references to: [email protected].
Structural and Functional studies of integrin signaling
My laboratory is interested in the molecular mechanisms underlying cell adhesion and motility mediated by integrin signaling and small GTPase signaling. Integrins are cell adhesion molecules that transduce signals across the plasma membrane (PM) bi-directionally. Misregulation of integrin expression and its activity may result in severe diseases such as autoimmune diseases, thrombotic disorders, cardiovascular diseases (CVDs), and T cell proliferation defects. The bi-directional signaling property of integrins makes both extracellular and intracellular intervention possible for clinical benefits. Currently, the common integrin-intervention strategy is to antagonize extracellular ligand binding. These antagonistic agents have shown effects in treating thrombosis and multiple sclerosis. Nevertheless, due to the lack of specificity in recognizing integrin species and other unknown properties, serious adverse effects have been reported to associate with use of integrin antagonists. Alternatively, integrin activities can be well regulated by intracellular signaling proteins in a species-specific manner. Thus, targeting these intracellular proteins, alone or in combination with the antagonistic intervention, has the potential to achieve greater therapeutic outcomes by better differentiating integrin species and preventing unwanted signaling events.
To assess the binding specificity of talin with different species of integrins, we generated fusion proteins of the talin head with various integrin tails for crystallization. The talin head domain was fused to a peptide derived from the talin-binding NPxY motif of several integrin β species. The crystal structure of the integrin β2 peptide in complex with the talin head domain was determined (shown in purple). We compared the β2:talin structure with the β3:talin structure, which we previously reported, and proposed a "seesaw" model for the "FERM-folded" configuration of the talin head. In this model, the two groups of side chains may compete for interactions, resembling two modes (A and B) of a seesaw. While the overall FERM domain configuration remains intact in the structure of the β2:talin complex, the K401-D125 salt bridge, observed in the β3:THD structure, is disrupted in the β2:talin structure. Instead, a K306-E350 salt bridge and E269/Y270-K345 interactions are observed in the β2:talin structure (boxed). How does the talin head configuration impact its interaction with integrin-β? It is plausible that the flexibility of the C-terminal helix of the talin head determines the "off" rate in talin:integrin-β association. These findings shed light on the species-specificity of talin in recognizing integrins.
Alterations in the regulation or expression of integrins have been implicated in many human diseases including inflammatory disorders, cardiovascular diseases, and cancer. In cancer biology, altered expression of integrins has been linked to tumor cell proliferation, metastasis and survival due to their role as adhesion receptors. Hence the integrin signaling pathway has become an appealing target for cancer therapy. Integrins can be activated by extracellular ligand binding in an outside-in manner or by growth factor stimulation through an inside-out pathway. Although antagonistic inhibitors have been shown to reduce early tumor angiogenesis, the inconsistent effect remain major obstacles for anti-cancer drug development. Alternatively, Integrin activities may also be suppressed by blocking the specific interactions of intracellular elements in the inside-out signaling.
The main focus of my lab is to understand the structural basis of intermolecular complexes and intramolecular rearrangements that control integrin-mediated cell adhesion and motility. Understanding the structural details of each signaling event, particularly the protein-protein interactions involved in this pathway, is the key to developing next-generation inhibitors. We aim to elucidate the structural basis of cytosolic inter-molecular complexes and intra-molecular domain rearrangements that modify the integrin activation, to validate these specific interactions revealed in the crystal structures, and to assess their roles in integrin activation in biochemical and physiological contexts. Our lab employs a combination of X-ray crystallography, biochemical and biophysical assays, cell-based functional studies, computer modeling, and organic synthesis to accomplish these goals.
Project 1. Regulatory mechanisms of RIAM
RAP1-interacting adapter molecule (RIAM) mediates RAP1-induced integrin activation. This function requires the translocation of RIAM to the plasma membrane (PM). The RAS-association (RA) segment of the RA-PH module of RIAM interacts with GTP-bound RAP1 and the PH segment of the RA-PH module interacts with phosphoinositol 4,5 bisphosphate (PI(4,5)P2), thus driving RIAM to the PM. The interaction of RAP1 and the RA d but this interaction is inhibited by an N-terminal segment, IN, of RIAM. We reported structural basis for the autoinhibition of RIAM by an intramolecular interaction between the IN region (aa 27-93) and the RA-PH module. We solved the crystal structure of IN-RA-RH, which reveals that the “IN” segment associates with the RA segment and thereby suppresses RIAM:RAP1 association. This autoinhibitory configuration of RIAM can be released by phosphorylation at Tyr45 in the IN segment. We then found the PH:PI(4,5)P2 interaction is also autoinhibited by a conserved inter-molecular interface that masks the PIP2 binding site in the PH domains of RIAM. Our data indicate that phosphorylation of RIAM by Src family kinases disrupts this PH-mediated interface, unmasks the membrane PIP2-binding site, and promotes integrin activation. We further demonstrate that this process requires phosphorylation of Tyr267 and Tyr427 in the RIAM PH domain by Src. Our data reveal an unorthodox regulatory mechanism of small GTPase effector proteins by phosphorylation-dependent PM association, and provide new insights into the link between FAK/Src kinases and integrin signaling.
Project 2. Active form of talin head
Binding of the intracellular adapter proteins talin and its cofactor, kindlin, to the integrin receptors induces integrin activation and clustering. These processes are essential for cell adhesion, migration, and organ development. Although the talin head, the integrin-binding segment in talin, possesses a typical FERM-domain sequence, a truncated form has been crystallized in an unexpected, elongated form. Our crystal structure of a full-length talin head in complex with the β3-integrin tail reveals a compact FERM-like conformation and a tightly associated N-P-L-Y motif of β3-integrin. A critical C-terminal poly-lysine motif, which is truncated in the linear form, mediates FERM interdomain contacts and assures the tight association with the β3-integrin cytoplasmic segment. Therefore, structural characterization of the FERM-folded active talin head provides fundamental understanding of the regulatory mechanism of integrin function, and may lead to rediscovery of the structural basis for the talin head-mediated signal transduction.
Project 3. Structural and functional studies of Kindlin.
The kindlin family includes three members, kindlin-1, kindlin-2, and kindlin-3. Kindlin possess a FERM domain similar to talin head, and a PH domain. While the β1 and β3 integrin CTs are the common binding partners of Kindlins, each Kindlin targets a distinct integrin β tail. For instance, kindlin-3 interacts with β2 integrin. In the keratinocytes, only Kindlin-1, but not Kindlin-2, can interact with β6 integrin. Kindlin-2 recognizes β7 integrin, but not β2 or β6 integrin in vitro. We have determined the crystal structure of the complete FERM domain of kindlin-2, with the PH domain removed for crystallization purpose (K2DPH). The structure revealed a canonical FERM domain architecture, in which the F1 domain sits on top of the F2F3 domains. Compared with the published kindlin-2 structure (PDB code: 5XPY), our structure affords the full-length F1 subdomain, allowing us to obtain more structural detail of the F1 loop, which is required for kindlin-mediated integrin co-activation. Moreover, we have obtained preliminary electron microscopic data for kindlin-2. Our data reveal a monomeric model for kindlin-2. Further analyses of the EM models and the oligomerization states of kindlin isoforms are required to understand how oligomerization impacts its activity.
Project 4. Regulatory mechanisms of talin by intramolecular interactions
Talin possesses a long, multi-domain, C-terminal “rod” region. Talin rod domains are helical bundle domains and regulate talin function by interacting the talin head domain and other signaling partners. Talin head interacts with the R9 domain in the rod region. To assess the possible intramolecular interactions between the rod domain adjacent to R9 and talin head, we generated a construct for the R9R10 tandem domains and determined its crystal structure. Both R9 and R10 domains are 5-helical bundles. Interestingly, the structure reveals that the last a-helix the R9 domain and the first a-helix of the R10 domain join together and form an extended helix that bridges the two domains (Figure 5A). Moreover, superposition of the R9R10 tandem domain and the R9:F2F3 autoinhibitory complex reveals potential interactions between the R10 and F3 domain (Figure 5A). In particular, Asp1943 of R10 is in close proximity to Lys364 of F3 with a distance of 5.6-Å in the superimposed models. Our data suggest that the R10 domain may also contribute to the head:rod autoinhibitory interaction. Further analyses are underway to validate this model.
In an effort to elucidate the intramolecular interaction in talin between the R1R2R3 triple domains and the F2F3 domains in the head region, we expressed and purified R1R2R3 triple domains. Previously reported NMR structures of the R3 domains revealed two different helical-bundle arrangements (PDB codes: 1U89 and 2L7A). 1U89 is composed of residues 755-889 (a1-a4), whereas 2L7A is composed of residues 788-911 (a2-a5) (Figure 2B). Although the two helical-bundles can be superimposed nicely, a1 helix in 1U89 is replaced by a5 in 2L7A. Moreover, it has been proposed that the R1R2R3 domains may form a rather compact module instead of a “beads-on-a-string” architecture. We have obtained crystals for R1R2R3 and are in the process of determining the structure of this triple domains (Figure 5C). Our result will yield essential insight to these questions.
Project 5. Species-specific interaction of talin and integrin
To assess the binding specificity of talin with different species of integrins, we generated fusion proteins of the talin head with various integrin tails for crystallization. The talin head domain was fused to a peptide derived from the talin-binding NPxY motif of several integrin β species. The crystal structure of the integrin β2 peptide in complex with the talin head domain was determined (shown in purple). We compared the β2:talin structure with the β3:talin structure, which we previously reported, and proposed a "seesaw" model for the "FERM-folded" configuration of the talin head. In this model, the two groups of side chains may compete for interactions, resembling two modes (A and B) of a seesaw. While the overall FERM domain configuration remains intact in the structure of the β2:talin complex, the K401-D125 salt bridge, observed in the β3:THD structure, is disrupted in the β2:talin structure. Instead, a K306-E350 salt bridge and E269/Y270-K345 interactions are observed in the β2:talin structure (boxed). How does the talin head configuration impact its interaction with integrin-β? It is plausible that the flexibility of the C-terminal helix of the talin head determines the "off" rate in talin:integrin-β association. These findings shed light on the species-specificity of talin in recognizing integrins.
Two modes of talin: integrin association. Mode A & B are represented by the 3 bound THD(purple) and the 2 bound THD (green), respectively. The F1:F3 (left) and F2: F3(right) interfaces are indicated by dashed boxes, with side chain interactions that stabilize the two modes showed in the enlarged boxes.
Related publications:
https://pubmed.ncbi.nlm.nih.gov/37078342/
https://pubmed.ncbi.nlm.nih.gov/33288722/
Project 6. Peptidomimetic inhibitor of talin-mediated integrin activation.
We have designed peptidomimetic inhibitors to competitively blocking talin binding to both RIAM and integrin, thus inhibiting integrin activation. We determined crystal structure of the peptidomimetic (S-TBS) with talin rod, which will help optimizing the peptide sequence and staple type to enhance its membrane permeability and affinity to talin. The inhibitory effect of S-TBS on integrin activity has been confirmed. Mutations/modifications will be introduced in the peptide to enhance its interaction with talin based on the structures. Optimized peptides will be re-tested for binding properties, membrane permeability, and inhibitory effect. The goal is to develop and optimize the new form of peptidomimetic reagents to inhibit integrin activity more specifically with less adverse effects.
Related publications:
https://pubmed.ncbi.nlm.nih.gov/37369205/
https://pubmed.ncbi.nlm.nih.gov/25520155/
https://pubmed.ncbi.nlm.nih.gov/25465129/
Gao T, Cho EA, Zhang P, Wu J. Inhibition of Talin-induced Integrin Activation by a Double-hit Stapled Peptide. Structure. 2023 Aug 3;31(8):948-957.e3. doi: 10.1016/j.str.2023.05.016. Epub 2023 Jun 26. PMID: 37369205.
Cho E.A., Zhang P., Kumar V., Kavalchuk M., Zhang H., Huang Q., Duncan J.S., Wu J., Phosphorylation of riam by src promotes integrin activation by unmasking the ph domain of riam. Structure. 29(4): 320-329 e4, 2021. PMC8026550. https://www.ncbi.nlm.nih.gov/pubmed/33275877.
Su B.,Wu J., Phosphorylation of riam activates its adaptor function in mediating integrin signaling. J Cell Signal. 2(2): 103-110, 2021. PMC8813058. https://www.ncbi.nlm.nih.gov/pubmed/35128538.
Zhang P, Azizi L, Kukkurainen S, Gao T, Baikoghli M, Jacquier MC, Sun Y, Maatta JAE, Cheng RH, Wehrle-Haller B, Hytonen VP, Wu J. Crystal structure of the FERM-folded talin head reveals the determinants for integrin binding. Proc Natl Acad Sci U S A. 2020. Epub 2020/12/09. doi: 10.1073/pnas.2014583117. PubMed PMID: 33288722.
Kukkurainen S, Azizi L, Zhang P, Jacquier MC, Baikoghli M, von Essen M, Tuukkanen A, Laitaoja M, Liu X, Rahikainen R, Orlowski A, Janis J, Maatta JAE, Varjosalo M, Vattulainen I, Rog T, Svergun D, Cheng RH, Wu J, Hytonen VP, Wehrle-Haller B. The F1 loop of the talin head domain acts as a gatekeeper in integrin activation and clustering. J Cell Sci. 2020;133(19). Epub 2020/10/14. doi: 10.1242/jcs.239202. PubMed PMID: 33046605.
Cho EA, Zhang P, Kumar V, Kavalchuk M, Zhang H, Huang Q, Duncan JS, Wu J. Phosphorylation of RIAM by Src Promotes Integrin Activation by Unmasking the PH Domain of RIAM. Structure. 2020. Epub 2020/12/05. doi: 10.1016/j.str.2020.11.011. PubMed PMID: 33275877.
Chang YC, Su W, Cho EA, Zhang H, Huang Q, Philips MR, Wu J. Molecular basis for autoinhibition of RIAM regulated by FAK in integrin activation. Proc Natl Acad Sci U S A. 2019;116(9):3524-9. doi: 10.1073/pnas.1818880116. PubMed PMID: 30733287; PMCID: PMC6397589.
Zhang H, Chang YC, Huang Q, Brennan ML, Wu J. Structural and Functional Analysis of a Talin Triple-Domain Module Suggests an Alternative Talin Autoinhibitory Configuration Structure. 2016 24(5):721-9. PubMed
Yang J, Zhu L, Zhang H, Hirbawi J, Fukuda K, Dwivedi P, Liu J, Byzova T, Plow EF, Wu J**, Qin J**. Conformational activation of talin by RIAM triggers integrin-mediated cell adhesion. Nat Comm Epub 2014 Dec 18. PubMed
Chang YC, Zhang H, Brennan ML, Franco-Barraza J, Patel T, Cukierman E, Wu J. Structural and mechanistic insights into the recruitment of talin by RIAM in integrin signaling. Structure 2014 22(12):1810-20. PubMed
Zhang H, Chang YC, Brennan ML, Wu J. The structure of Rap1 in complex with RIAM reveals specificity determinants and recruitment mechanism. J Mol Cell Biol 2014 6(2):128-39. PubMed
Anastassiadis T, Duong-Ly KC, Deacon SW, Lafontant A, Ma H, Devarajan K, Dunbrack RL Jr, Wu J, Peterson JR. A highly selective dual insulin receptor (IR)/insulin-like growth factor 1 receptor (IGF-1R) inhibitor derived from an extracellular signal-regulated kinase (ERK) inhibitor. J Biol Chem. 2013 Sep 27;288(39):28068-77. doi: 10.1074/jbc.M113.505032. Epub 2013 Aug 9. PubMed
Chang YC, Zhang H, Brennan ML, Wu J. Crystal structure of Lamellipodin implicates diverse functions in actin polymerization and Ras signaling. Protein Cell. 2013 Mar;4(3):211-9. doi: 10.1007/s13238-013-2082-5. Epub 2013 Mar 13. PubMed
Wynne JP*, Wu J*, Su W, Mor A, Patsoukis N, Boussiotis VA, Hubbard SR, Philips MR. Rap1-interacting adapter molecule (RIAM) associates with the plasma membrane via a proximity detector. J Cell Biol. 2012 Oct 15;199(2):317-30. doi: 10.1083/jcb.201201157. Epub 2012 Oct 8. *Equal contribution. PubMed
Ungureanu D, Wu J, Pekkala T, Niranjan Y, Young C, Jensen ON, Xu CF, Neubert TA, Skoda RC, Hubbard SR, Silvennoinen O. The pseudokinase domain of JAK2 is a dual-specificity protein kinase that negatively regulates cytokine signaling. Nat Struct Mol Biol. 2011 Aug 14. [Epub ahead of print] PubMed
Depetris RS*, Wu J*, Hubbard, S.R. Structural and functional studies of the Ras-associating and pleckstrin-homology domains of Grb10 and Grb14. Nat Struct Mol Biol. 2009;16,833-9. *Equal contribution. PubMed
Wu J*, Li W*, Craddock BP, Foreman KW, Mulvihill MJ, Ji QS, Miller WT, Hubbard SR. Small-molecule inhibition and activation-loop trans-phosphorylation of the IGF1 receptor. EMBO J. 2008;27,1985-94. *Equal contribution. PubMed
Wu J, Tseng Y, Xu C, Neubert TA, White MF, and Hubbard SR. Structural and biochemical characterization of the KRLB region in insulin receptor substrate-2. Nat Struct Mol Biol. 2008;15,251-8. PubMed
A postdoctoral position is available in the Wu lab at Fox Chase Cancer Center in the field of structural biology and protein biochemistry. Teh Wu lab focuses on structural and mechanistic studies of the signaling events mediated by integrins, small GTPases, and kinases, as well as development of inhibitor and/or drug that target these events. The lab is equipped with state-of-the-art instruments, including a Mosquito robot, a Formulatrix crystal imager, and a brand-new Synergy-S X-ray home source. We also have frequent beamtimes at BNL and MacChess synchrotrons. The ideal candidate for the position should hold a Ph.D. with strong expertise in X-ray crystallography and are also expected to be experienced in construct design, protein expression and purification. Prior experience in mammalian cell culture, cell signaling, flow cytometry, and cancer biology is desired but not required.
As one of the four original cancer centers to receive comprehensive designation from the National Cancer Institute, Fox Chase Cancer Center has been at the forefront of cancer research for almost 90 years. We are home to excellent research facilities, top clinicians and scientists, and outstanding patient care. Our singular focus on cancer, which couples discovery science with state of the art clinical care and population health, remains the foundation of our work.
The scientist training programs at Fox Chase Cancer Center provide professional development opportunities in four core areas identified as crucial for successful careers in science, research, and health care including communication, leadership, teaching, and mentorship. Upon joining the program, graduate students and postdocs develop individual development plans to help guide their growth. Training throughout the year is supplemented with free professional development opportunities, including a robust ‘How To’ series, writing courses, networking, mentorship, and teaching opportunities, a trainee-led seminar series, a trainee-led annual Research Conference, and more. Postdocs at Fox Chase Cancer Center are supported by the Temple University Postdoc Association and the Office of Academic Affairs at Fox Chase, and are compensated with competitive pay and benefits.
In addition to the robust training program, scientists at Fox Chase Cancer Center benefit from being part of the rich scientific and biotech environment in the Philadelphia region. Many of our former trainees are now employees (and contacts) at nearby institutions and companies, including The Wistar Institute, Merck, GSK, AACR, and numerous others.
If you are interested in the position or have any questions regarding the position, please contact Dr. Jinhua Wu ([email protected]. To apply for the position, please send a cover letter describing research experience and future plans, a current CV, and a list of three references.
The following ratings and reviews are based on verified feedback collected from independently administered patient experience surveys. The ratings and comments submitted by patients reflect their own views and opinions. Patient identities are withheld to ensure confidentiality and privacy. Learn more about our Patient Experience Ratings.
This Fox Chase professor participates in the Undergraduate Summer Research Fellowship.
Learn more about Research Volunteering.
Patient comments